The formation of neural circuitry is an astonishing feat. Within a matter of months, two individual cells transform into a fully functioning nervous system. By adulthood, a single cubic millimeter of human brain tissue, roughly the size of a sharpened pencil tip, contains over 150 million connections.1 But how does this incredibly complex organ develop? What are the levers that kickstart and propel this developmental cascade forward? How sensitive are these neurobiological processes to environmental influence? How much neural development occurs before versus after birth? Are individual differences in the initial development of neural phenotypes predictive of future health and disease?
My work, and the work of others, seeks to address these pressing scientific questions using fetal and infant magnetic resonance imaging (MRI). MRI is a noninvasive technology that leverages a strong magnetic field to estimate brain activity via changes in blood oxygen level, reconstruct detailed images of human anatomy, and quantify white matter properties such as myelination. Although commonly used in adults, adolescents, and children, MRI has only recently been used to study infant and fetal brain development. Many of the same analytic methods that are used in postnatal MRI can be applied to fetal MRI, including quantitative analysis of white matter tracts, resting-state functional connectivity analyses, and volumetric analysis of brain structure. The major difference between fetal and postnatal MRI lies in image processing. That is, fetal MRI data tends to contain higher levels of noise and thus requires specialized processing techniques. In addition to motion-related noise inherent to scanning very young ages, in vivo fetal MRI can be impacted by noise from maternal anatomy and lower signal-to-noise ratio since you are imaging the brain while it is inside the maternal compartment. These challenges have historically prevented widespread use of fetal and infant MRI. However, technological advances have made huge strides in overcoming these challenges, making it easier to collect and analyze infant and fetal MRI data than ever before. In addition to automated and semi-automated processing pipelines, fetal and infant MRI datasets are publicly available for researcher use. For example, the Developing Human Connectome Project (dHCP) issued their fourth data release earlier this year, which includes minimally processed functional, diffusion, and structural MRI data from 255 human fetuses and 809 human newborns at the time of this writing.2
In honor of this exciting data release, I wanted to highlight a few compelling scientific discoveries that have been made possible by fetal MRI in particular.
The beginnings of adult-like functional networks are in place prior to birth
The first paper on fetal resting-state functional MRI was conducted in only 16 fetuses that spanned 20 to 36 gestational weeks. Yet even at this young age, and prior to birth, two analogs of major resting-state networks were detected – a bilateral frontal network and a bilateral visual network.3 Identification of proto visual networks in the fetal brain is particularly remarkable given extremely limited, if any, visual input prior to birth. Since this first paper, analogs of other major canonical resting-state networks that are present postnatally have been detected in a larger fetal cohort4 and in preterm-born infants,5 including auditory, sensorimotor, default mode, and subcortical proto networks. In addition to identifying the presence of early networks, graph theory has been used to identify hubs, or highly connected regions, in the fetal brain. Interestingly, two major hubs that emerged from this analysis were bits of cortex that become specialized for perceiving faces and understanding language by adulthood.6 This finding raises the intriguing possibility that humans may come into the world prepared to process important social cues.
These studies do not suggest neural circuitry is fully formed at birth, despite significant overlap in fetal and adult connectomes.7 Rather, there is dramatic, nonlinear change in brain structure and function across gestation and into the postnatal period. For example, at least one study identified an inflation point in whole-brain functional connectivity metrics at 25-28 gestational weeks. Prior to this inflation point, mean functional connectivity values were low for resting-state networks. After 25-28 gestational weeks, rate of change in connectivity values increased by more than 90%, suggesting the third trimester is a key ‘expansion period’ for functional network development.8 Functional specialization, network restructuring, and anatomical changes continue into the postnatal period, with widespread changes in brain structure evident across infancy, childhood, adolescence, and even into adulthood.9,10 Nonetheless, the fetal studies collectively suggest that the way we respond to the environment may be influenced by neural circuitry that is in place prior to birth. Such a possibility, and fetal MRI as a tool, open the door for critically important studies that will determine whether proto resting-state networks require experience to develop associated functions, and whether there are critical periods when structure/function mapping may be most primed to occur.
Fetal connectivity patterns correlate with infant and toddler behavior
Whether neural phenotypes early in life are informative for predicting future behavior is of immense clinical significance. To date, few human studies have prospectively linked individual differences in fetal neural connectivity with postnatal behavior. Yet studies are beginning to address this gap.
Using functional MRI data from 120 fetuses, Ji and colleagues showed that spontaneous coactivation of the supplementary motor area with visual cortex and other posterior brain regions in utero correlated with future motor behavior.11 Specifically, more frequent supplementary motor area to posterior cortex co-activation associated with more mature motor behavior at 7 months postpartum. In addition, some of my own work has shown that neural correlates of emotion dysregulation are detectable in utero, well before the onset of clinical symptomatology. In this study (n=79), functional coupling between frontolimbic regions in the fetal brain correlated with future emotion dysregulation and aggressive behaviors at 3 years of age, even after accounting for co-occurring pre- and postnatal exposure to low income, maternal stress, maternal depression, and maternal anxiety.12 These preliminary findings, along with others, underscore the behavioral, and potentially clinical, significance of individual differences in prenatal brain development. Excitingly, the new dHCP data release includes measures of infant behavior, so it will be possible for independent research groups to replicate and extend findings that tie in utero neural connectomics to future behavior.
Conclusions and future directions
Because fetal MRI allows examination of the initial wiring of neurocircuitry, this tool is well-poised to provide direct insight into the very foundations of human brain development and etiology of neurodevelopmental disorders. Examination of fetal brain development inherently occurs prior to postnatal exposures, such as parenting, that we know influence child growth and behavior. Thus, fetal MRI also enables researchers to disentangle impact of the gestational environment from postnatal exposures, with potential to isolate sensitive windows for exposure impact. Combined with genetically informed research designs, fetal MRI may eventually be used to answer fundamental questions about the extent to which neural phenotypes result from nature versus nurture.
References
- Shapson-Coe A, Januszewski M, Berger DR, et al. (2024) A petavoxel fragment of human cerebral cortex reconstructed at nanoscale resolution. Science, 384(6696). DOI: https://doi.org/10.1126/science.adk4858.
- Karolis, V., Cordero-Grande, L., Price, A. N., et al. (2024). The developing Human Connectome Project fetal functional MRI release: Methods and data structures. bioRxiv Preprint, DOI: https://doi.org/10.1101/2024.06.13.598863
- Schöpf, V., Kasprian, G., Brugger, P. C., & Prayer, D. (2012). Watching the fetal brain at ‘rest’. International Journal of Developmental Neuroscience, 30(1), 11-17. DOI: https://doi.org/10.1016/j.ijdevneu.2011.10.006.
- Ji, L., Hendrix, C. L., & Thomason, M. E. (2022). Empirical evaluation of human fetal fMRI preprocessing steps. Network Neuroscience, 6(3), 702-721. DOI: https://doi.org/10.1162/netn_a_00254.
- Doria, V., Beckmann, C. F., Arichi, T., et al. (2010). Emergence of resting state networks in the preterm human brain. Proceedings of the National Academy of Sciences, 107(46), 20015-20020. DOI: https://doi.org/10.1073/pnas.100792110
- van den Heuvel, M. I., Turk, E., Manning, J. H., et al. (2018). Hubs in the human fetal brain network. Developmental Cognitive Neuroscience, 30, 108-115. DOI: https://doi.org/10.1016/j.dcn.2018.02.001
- Turk, E., Van Den Heuvel, M. I., Benders, M. J., et al. (2019). Functional connectome of the fetal brain. Journal of Neuroscience, 39(49), 9716-9724. DOI: https://doi.org/10.1523/JNEUROSCI.2891-18.2019.
- Jakab, A., Schwartz, E., Kasprian, G., et al. (2014). Fetal functional imaging portrays heterogeneous development of emerging human brain networks. Frontiers in Human Neuroscience, 8. DOI: https://doi.org/10.3389/fnhum.2014.00852.
- Rutherford, S., Fraza, C., Dinga, R., Kia, S. M., et al. (2022) Charting brain growth and aging at high spatial precision. eLife, 11(e72904). DOI: https://doi.org/10.7554/eLife.72904.
- Bethlehem, R.A.I., Seidlitz, J., White, S.R. et al. Brain charts for the human lifespan (2022). Nature, 604. https://doi.org/10.1038/s41586-022-04554-y.
- Ji, L., Majbri, A., Hendrix, C. L., & Thomason, M. E. (2023). Fetal behavior during MRI changes with age and relates to network dynamics. Human Brain Mapping, 44(4), 1683-1694. DOI: https://doi.org/10.1002/hbm.26167
- Hendrix, C. L., Ji, L., Werchan, D. M., et al. (2023). Fetal frontolimbic connectivity prospectively associates with aggression in toddlers. Biological Psychiatry Global Open Science, 3(4), 969-978. DOI: https://doi.org/10.1016/j.bpsgos.2022.09.003
About the Author
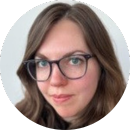
Cassandra L. Hendrix, PhD
Department of Psychology, University of Hawai’i Mānoa
Casandra Hendrix is an Assistant Professor at the University of Hawai’i Mānoa. Her research investigates the developmental origins of psychopathology. She is particularly interested in isolating perinatal mechanisms by which adversity is passed from parents to their children. In this work, she integrates fetal and infant magnetic resonance imaging with behavioral assessments, diagnostic evaluations, and measures of stress physiology across pregnancy and the postpartum. By understanding early environmental, biological, and behavioral precursors of stress-related psychopathology, she hopes to illuminate its cascading etiology and inform preventative interventions. Her work has been funded by NSF, the American Psychological Foundation, and a Pathway to Independence Award from NIMH.